Roberto MARQUARDT
Professor
✉️ roberto.marquardt[at]unistra.fr
STUDENTS:
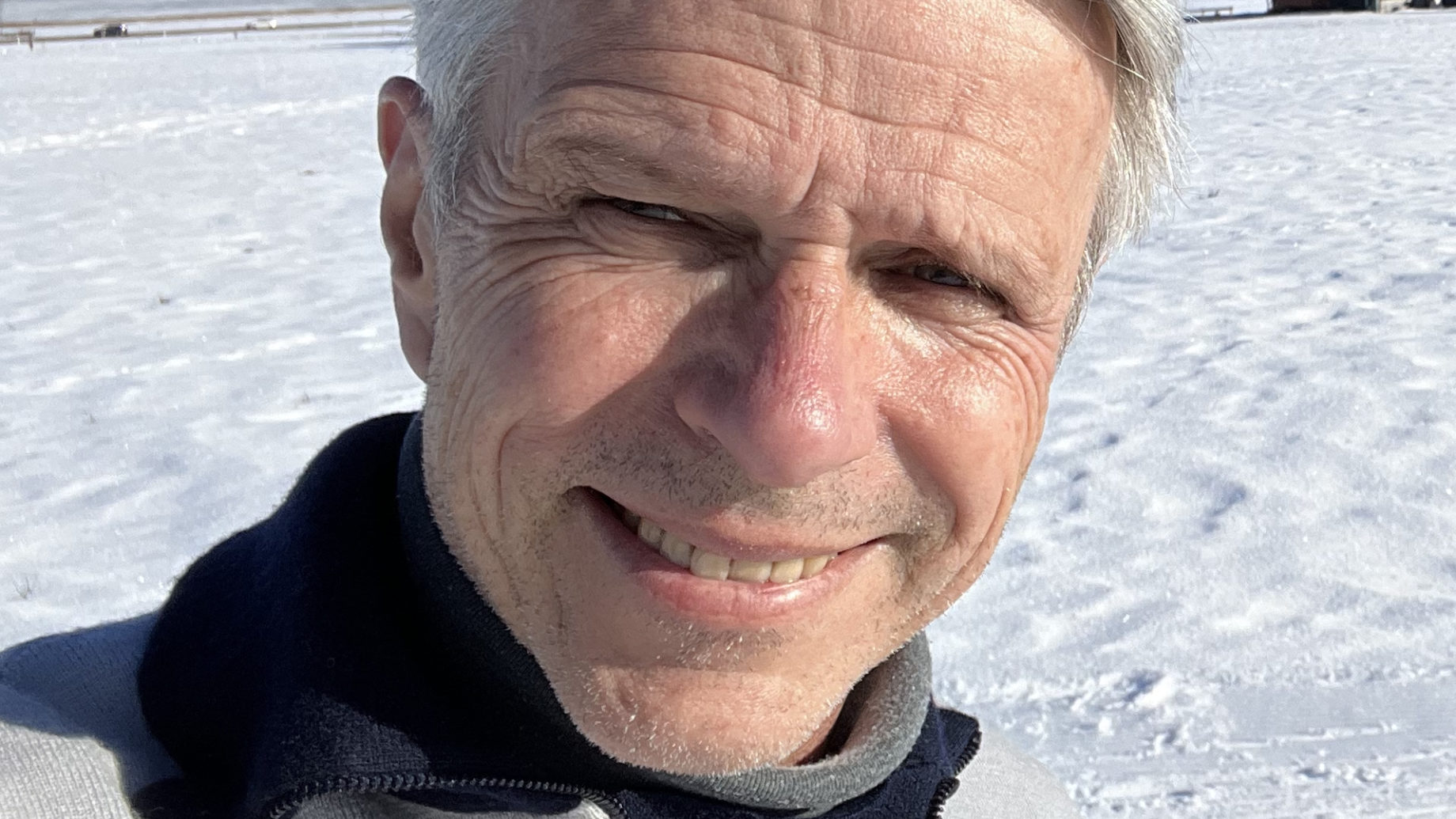
Complete list of my publications: .pdf
Research Topics
Roberto Marquardt’s research interests has been in the field of molecular quantum dynamics, in particular the dynamics of highly excited vibrational states and the related high resolution spectroscopy of small molecular compounds. The list of publications reflects the research activities. More recent work addresses their quantum dynamics at the gas-solid interface as well as electronically excited states. A brief synopsis of current research activities is presented in the following.
The motion of adsorbates on crystalline surfaces
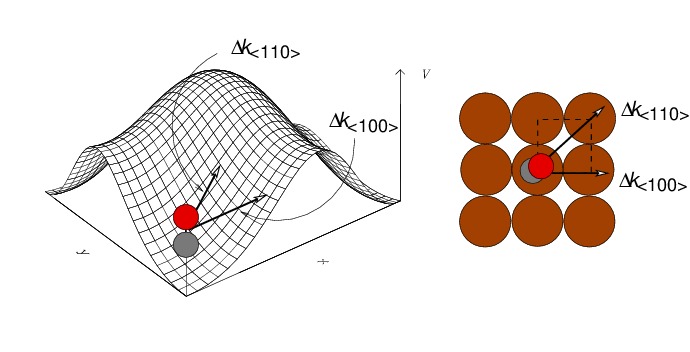
Heterogeneous catalysis is an essential process for the world’s economy and its sus-tainable growth. While progress has been made in the past decades to its under-standing, many elementary steps remain unresolved. One very important step is the diffusion of the chemically reacting adsorbates on the catalytic substrate. The nature of this elementary step has been unraveled since more than 20 years by high resolution 3He-spin-echo experiments. The interpretation of these experiments remains controversial. For instance, the barrier for diffusion of CO on a copper surface is predicted to be three times higher along the <110> direction than along the <100> direction [1], as indicated in the figure below, whereas the spin echo experiments seem to indicate that the barriers should be similar (Alexandrowicz et al. Phys. Rev. Lett. 93, 156103 (2004)).
Insight into the experimental outcome was gained from first principle calculations [2], for the very first time, showing that quantum effects are important above room temperature. The theory developed in that work allowed us for instance to calculate diffusion rates α for H and H2 on Pd(111), yielding significant differences. Is it H or H2 that is being monitored in the experiments? The answer to this question is not obvious at all.
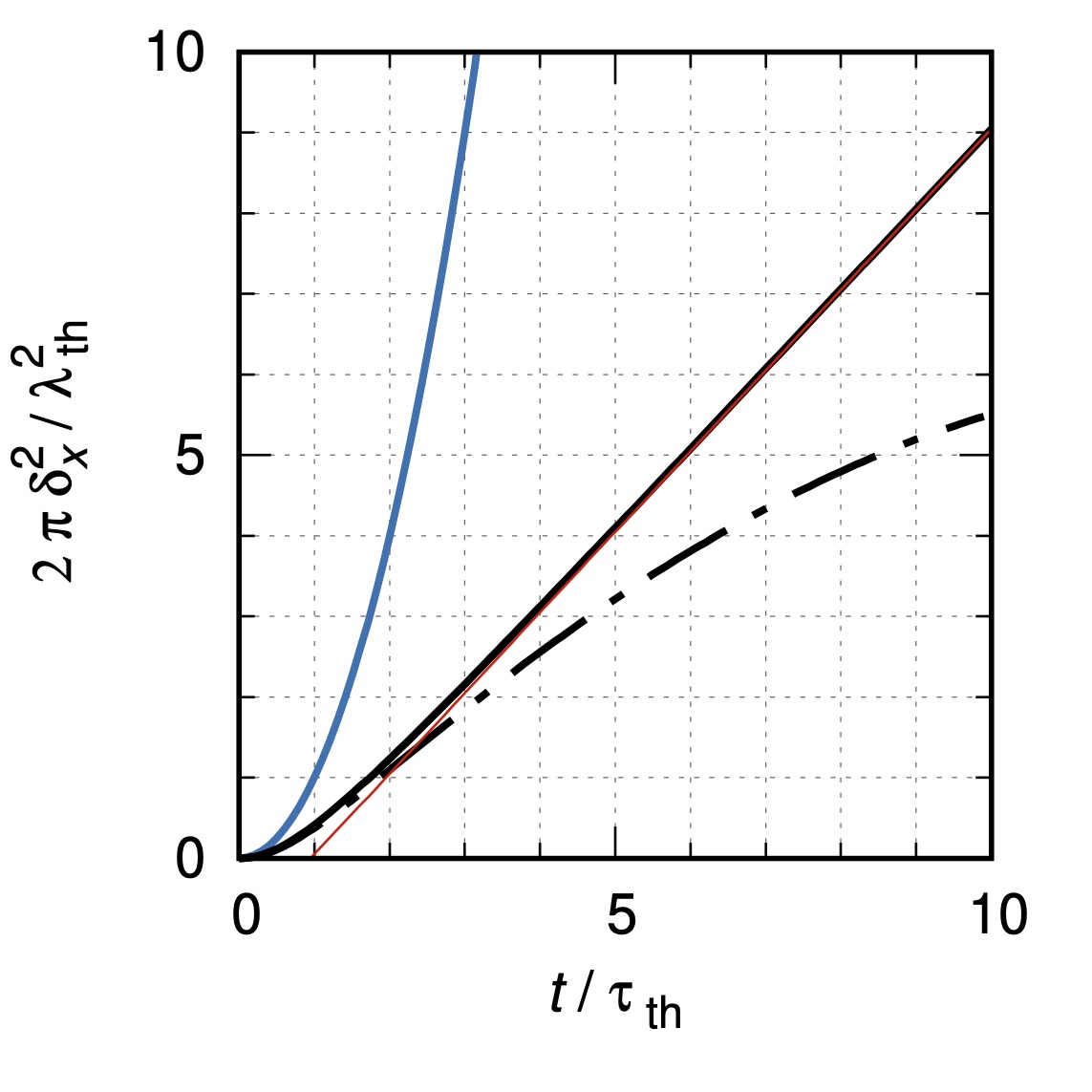
In the course of these investigations, the mean square displacement (MSD) of a thermalized particle, a key quantity in statistical mechanics, was investigated from the quantum mechanical prospective [3–6]. The figure below shows the MSD of a free particle of mass m moving on a ring following the so-called thermal wave packet definition [3]. The interrupted black line is for a finite ring of length –
is the so-called thermal de Broglie wave length at temperature T . The continuous black line is for an infinitely long ring, the red line represents the asymptotic limit
. The blue line is the expected classical result for ballistic motion. The time unit
is the so-called thermal time.
This result is an interesting discrepancy between classical and quantum mechanics. Currently, a proper quantum mechanical definition of the MSD is pending [6].
- [1] R. Marquardt, F. Cuvelier, R. A. Olsen, E. J. Baerends, J. C. Tremblay, and P. Saalfrank. A New Analytical Potential Energy Surface for the Adsorption System CO/Cu(100). J. Chem. Phys., 132:074108, 2010. doi:10.1063/1.3308481.
- [2] Thiago Firmino, Roberto Marquardt, Fabien Gatti, and Wei Dong. Diffusion Rates for Hydrogen on Pd(111) from Molecular Quantum Dynamics Calculations. J. Phys. Chem. Lett., 5(24):4270–4274, 2014. doi:10.1021/jz502251w.
- [3] Roberto Marquardt. Mean square displacement of a free quantum particle in a thermal state. Molecular Physics, 119(17-18):e1971315, 2021. doi:10.1080/00268976.2021.1971315.
- [4] Roberto Marquardt. The quantum mean square displacement of thermalized CO on Cu(100) in the short time approximation. Phys. Chem. Chem. Phys., 24:26519, 2022. doi:10.1039/D2CP03045D.
- [5] Oussama Bindech and Roberto Marquardt. Mean square displacement of a free quantum particle on the basis of thermal Gaussian wave packets. Eur. J. Phys. Spec. Top.,2023. doi:10.1140/epjs/s11734-023-00920-7.
- [6] O. Bindech, F. Gatti, S. Mandal, R. Marquardt, L. Shi, and J. C. Tremblay. The mean square displacement of a ballistic quantum particle. Molecular Physics, 0(0):e2322023, 2024. doi:10.1080/00268976.2024.2322023.
The electronic spectroscopy of the dicopper complex
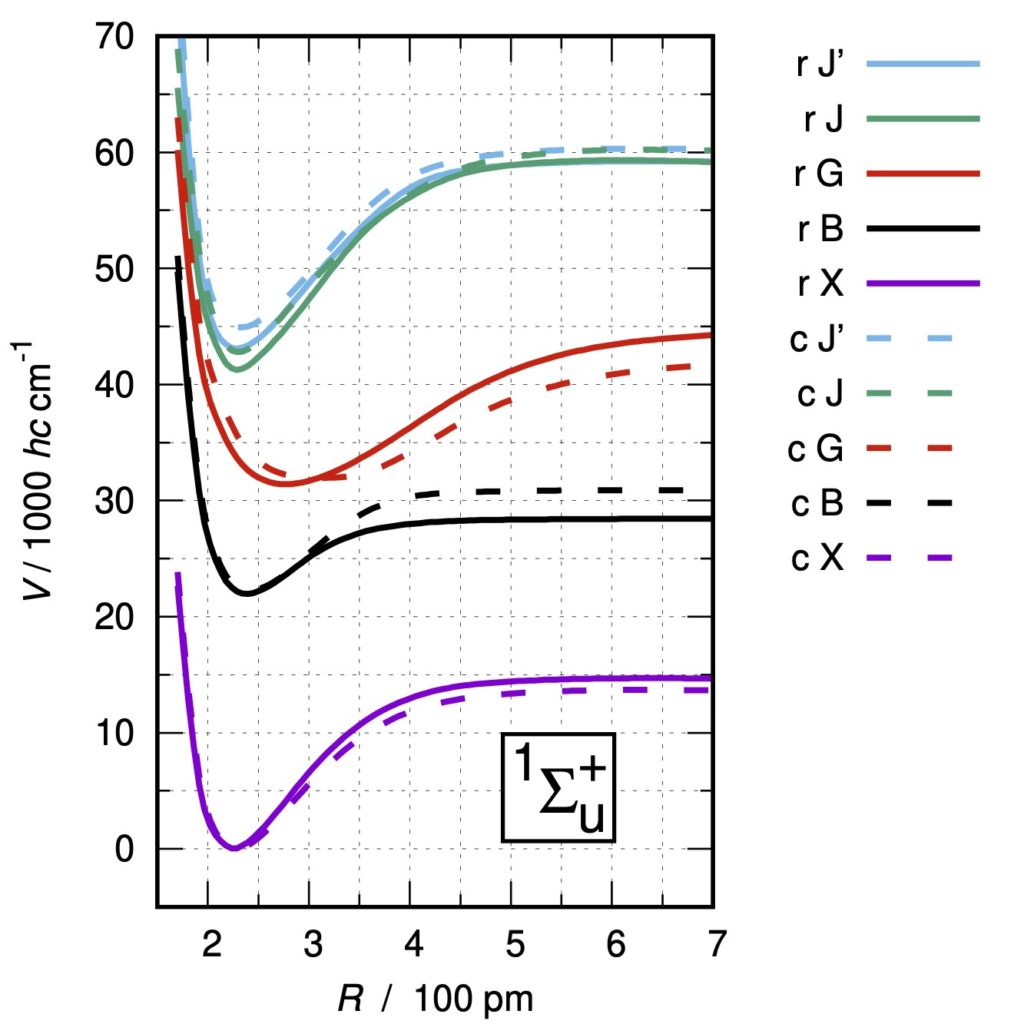
Electronically excited states of the Cu2 system has been studied at very high spectroscopic resolution with a resonant four wave mixing Laser spectroscopy at the Paul Scherrer Institute in Switzerland. With the assistance from ab initio calculations, an assignment problem could be solved that lasted for more than 40 decades [1]. Experiments and theory also lead to the determination of the currently most precise value of the dissociation energy of this prototype transition metal compound ((16 270 ± 7) hc cm−1 = (2.017 ± 0.001) eV) [2], in addition to the assignment of further highly excited states [3, 4]. The figure below shows the potential energy functions of some of these states. Relativistic corrections are essential as indicated by the non-corrected functions reproduced with dotted lines.
- [1] B. Visser, M. Beck, P. Bornhauser, G. Knopp, J. A. van Bokhoven, R. Marquardt, C. Goulaouen, and P. P. Radi. Identification of a new low energy 1u state in dicopper with resonant four-wave mixing. J. Chem. Phys., 147:214308, 2017. doi:10.1063/1.5006107.
- [2] P. Bornhauser, M. Beck, Q. Zhang, G. Knopp, R. Marquardt, C. Gourlaouen, and P. P. Radi. Accurate ground state potential of Cu2 up to the dissociation limit by perturbation assisted double-resonant four-wave mixing. J. Chem. Phys., 153(24):244305, 2020. doi:10.1063/5.0028908.
- [3] Jiaye Jin, Qiang Zhang, Peter Bornhauser, Gregor Knopp, Roberto Marquardt, and Peter P. Radi. Rovibrational investigation of a new high-lying 0+u state of Cu2 by using two-color resonant four-wave-mixing spectroscopy. The Journal of Chemical Physics, 156(18):184305, 2022. arXiv:https://doi.org/10.1063/5.0087743, doi:10.1063/5.0087743.
- [4] Q. Zhang, P. Bornhauser, G. Knopp, P.P. Radi, G. Harmant, and R. Marquardt. Experimental and theoretical investigation of excited g-symmetry states of Cu2 . Chemical Physics Letters, 803:139822, 2022. doi:10.1016/j.cplett.2022.139822.
Molecular quantum dynamics of isolated molecules and under the influence of coherent radiation
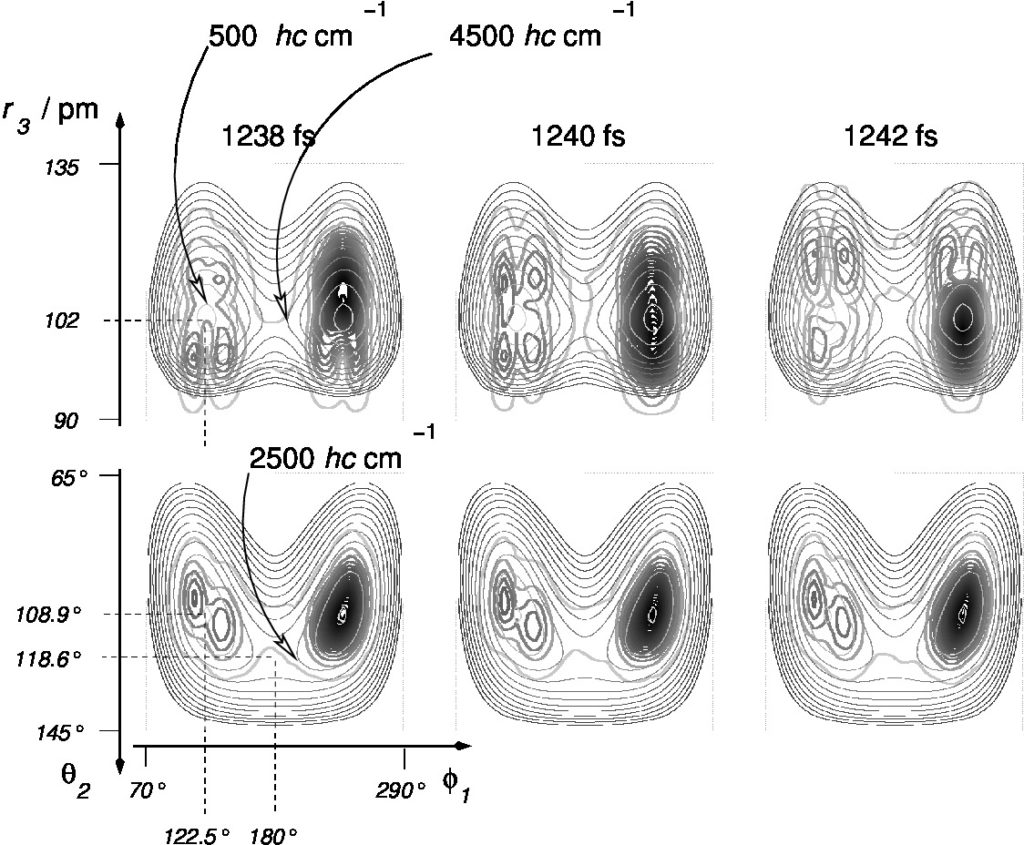
Understanding the motion of atoms in molecules is essential for the understanding of chemical reactions. The motion is best described in terms of time evolving probability densities representing the time dependent distribution of atomic positions in nuclear configuration space. The figure below shows contour lines of the probability density for finding planar ammonia molecules after infrared excitation of the NH stretching vibration in the ammonia isotopologue NHD2 [1].
Full (12-dimensional) theoretical simulations have also shown how coherent infrared radiation can be used to enhance or inhibit tunneling motion in ammonia [2].
The quantum dynamics of ammonia, methane and other molecules was addressed in numerous articles given in the full list of references.
- [1] R. Marquardt, M. Sanrey, F. Gatti, and F. Le Quéré. Full-Dimensional Quantum Dynamics of Vibrationally highly excited NHD2 . J. Chem. Phys., 133:174302, 2010. doi:10.1063/1.3496374.
- [2] Csaba Fábri, Roberto Marquardt, Attila G. Császár, and Martin Quack. Controlling tunneling in ammonia isotopomers. The Journal of Chemical Physics, 150(1):014102, 2019. doi:10.1063/1.5063470.